
A new method for the evaluation of pelvic organ prolapse in women using a three- dimensional optic scanner
Article authors
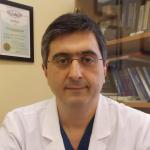
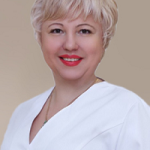
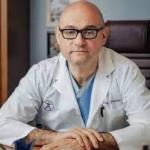
Abstract
Introduction and hypothesis Modern classifications of pelvic floor movements are based on pelvic floor assessment in a static midsagittal plane. This study presents a new and potentially useful 3D noninvasive tool for studying pelvic floor mobility in women with pelvic organ prolapse (POP).
Materials and methods Thirty-four patients with POP [grade ≥3 using the Pelvic Organ Prolapse Quantification (POP-Q) system] and 30 healthy volunteers (controls) at rest and during Valsalva maneuver were scanned using an Artec™ 3D optic portable scanner and 3D pelvic floor models were generated. We calculated the volume of the prolapsed vaginal wall using dynamic prolapse increment (DPI), which is defined as an increase in prolapse volume from rest to its maximal Valsalva probe [DPI= (Vval – Vrest) / Vrest %)].
Results In the control group, the average DPI was 28 % (16– 51 %). As the DPI in patients with POP varied widely, two subgroups were identified. In the first subgroup, the average DPI was 290 % (125–437 %), whereas it was only 48.8 % (41–55 %) in the second subgroup. Prolapse volume in subgroup 1 was not due to the most prominent component of POP but was induced by enlargement of the prolapsed vaginal wall from other components, such as a cystocele or enterocele, which was evident only during the 3D procedure and could not be validated by the POP-Q system.
Conclusions In addition to existing methods, 3D modelling is a useful tool for evaluating pelvic floor mobility. Further investigation of the pelvic floor dynamic features in women is necessary.
Introduction
Pelvic floor prolapse (POP) is a common condition that presents as the descent of pelvic floor organs. The bladder, urethra, uterus, vaginal vault, and posterior compartment (posterior vaginal wall, perineal body, rectum, anal sphincters) can change normal positions due to a subtle injury to the pelvic support system and can lead to progressive POP.
In contrast to modern POP classifications, only 3D pelvic floor reconstruction can identify defects not only in the midsagittal plane at the most prominent edge but in the entire pelvic floor. Numerical simulations provide various tools for studying pelvic floor function and can be used to explore the pathophysiology of POP by reproducing different levels of abnormalities or impairments in the pelvic floor support system that cannot be evaluated clinically [1–3]. Twodimensional and 3D models of POP have been created to simulate prolapse of the anterior vaginal wall and are mainly based on results of magnetic resonance imaging (MRI) [4, 5]. Current studies provided substantial evidence that is useful for POP diagnosis and treatment; however, computer simulations and compilations 2D images often oversimplify many aspects of pelvic support structures. In the majority of studies, 3D reconstruction is conducted on the basis of 2D images collected during MRI or ultrasound examinations.
The objective of our study was to introduce a new tool for evaluating andmeasuring pelvic floor mobility in womenwith POP.
Materials and methods
Artec Group LLC, Luxembourg). Structured illumination is the central principle of the scanning process. It is an optical method that allows digitization of a single frame of a sufficiently large surface area of a scanned object. The Artec 3D scanner (Fig. 1) delivers information regarding the shape of an object within a matter of seconds up to several minutes. As a result, this device is used whenever there is a need for 3D images of any body part or the entire human body. Being an optical device, the scanner presents zero safety risk to patients. Therefore, there is no need to determine or specify individual markers during the scanning procedure.
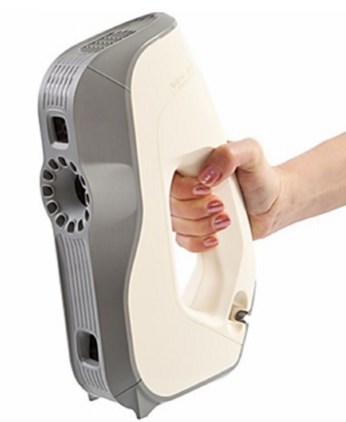
Fig. 1 Three-dimensional optic scanner (Eva Artec™, Artec Group LLC, Luxembourg)
The pelvic floor was scanned with a frequency of up to 16 frames per second for several minutes to obtain highdefinition (HD) images. A scan was performed with the patient in the lithotomy position and the equipment in the texture-scanning mode. Smooth movements were used at a distance, corresponding to the scanning range (40–100 cm) around the surface under the area of the site being studied: the vaginal walls and perineum. It is important to take images of the object from angles in directions from top to bottom and from left to right to digitize all areas not easily accessible due to light interference.
The study protocol was approved by the Local Ethical Committee. All participants signed an informed consent form and legal waivers. Thirty-four patients with severe symptomatic POP [stage 3–4 according to the Pelvic Organ Prolapse Quantification (POP-Q) classification] were studied. The volunteers were 30 randomized women ≥18 years with no history of POP, POP surgery, urinary incontinence (UI), or hysterectomy.
All women answered a set of questions regarding their anamnesis vitae of POP symptoms, urinary incontinence, constipation, chronic cough, and smoking habits. For imaging, all study participants were placed in the lithotomy position and given a set of instructions in a standardized way and instructed to stay at rest during the first scanning procedure and then to adopt a maximal straining position for 10 s. Both patients and volunteers underwent two immediate pelvic floor scan sessions: one at rest and another during maximal Valsalva. Patients were cautioned to avoid making random movements during scanning sessions, which could lead to false results. An assistant parted the patient’s labia, if necessary, to visualize the hymenal ring. If POP that extended past the hymenal ring at rest was present, a third additional scanning procedure was performed after preliminary manual repositioning of the POP during the first 10 s.
Data were treated with a registration-image alignment protocol preprogrammed into an Artec Studio application. Three-dimensional pelvic floor models at rest and straining were generated. The accepted plane of the hymenal ring as a reference for measurement was achieved by cropping images along the hymenal ring. The resulting models had a hollow shape, with the surface represented by vaginal walls, where the hymenal ring plane was a border. Subsequently, the same software calculated the surface area and volume of this geometric shape (Fig. 2). The total prolapse volume (TPV) was computed as the difference between prolapse volume during Valsalva and at rest. The dynamic prolapse increment (DPI) was calculated as a measure of TPV in relation to prolapse volume at rest: DPI = (Vval − Vrest) / Vrest ×100 %. The 8-min time interval was the mean duration of the scanning process and included information processing, model creation, and all calculations and measurements.
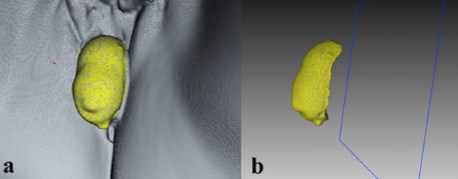
Fig. 2 Creating the 3D model (a) with calculated surface area and volume of the obtained geometric shape (b)
Results
Three-dimensional modelling of the pelvic floor was implemented for 34 patients with POP. The majority of study participants had anterior compartment prolapse (30/34) with/ without uterine prolapse. In some cases, there was a significant enterocele (8/34) or rectocele (2/34).
The DPI and TPV were calculated for all participants according to the methods described above. As soon as data were analyzed, it became apparent that at least two subgroups of patients could be identified. In the first subgroup (n=20), the DPI dramatically increased after Valsalva and reached a mean value of 290 % (125–437 %) (Fig. 3). Another group of patients with stage 3 or 4 POP-Q prolapse demonstrated a minor prolapse volume increment during Valsalva of 48.8 % (41– 55 %) (Fig. 4). Moreover, TPVs in the two subgroups did not differ significantly and were at 50.9 and 64.9 ccs for subgroups 1 and 2, respectively (Table 1).
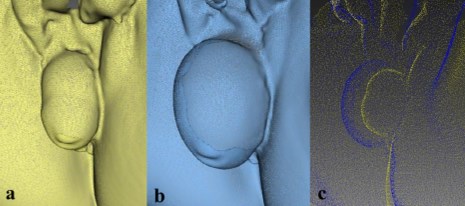
Fig. 3 Three-dimensional pelvic floor models in the first subgroup of pelvic organ prolapse (POPC) patients: at rest C(a), during straining (b), overlapping images at rest and during straining (c)
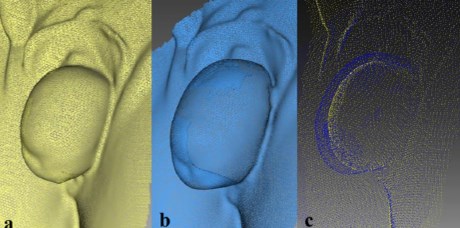
Fig. 4 Three-dimensional pelvic floor models in the second subgroup of pelvic organ prolapse (POP) patients: at rest (a), during straining ( b), overlapping images at rest and during straining (c)
Table 1 Characteristics of patients with pelvic organ prolapse (POP)
DPINumberAgeDeliveriesParitiesHysterectomyBMIPrevious POP surgery
POP 3–4 stageTotal3463.52.103.708/3431.24/34
DPI < 100 %2064.82.13.80/20310/20
DPI > 100 %1462.12.23.48/1430.54/14
POP pelvic organ prolapse, DPI dynamic prolapse Increment, BMI body mass index
The important point is that the increase in prolapse volume in subgroup 1 was not due to the most prominent component of POP (e.g., uterus) but induced by enlargement of the prolapsed vaginal wall due to other components, such as cystocele or enterocele. Using 3D POP reconstruction, several cases of asymmetric damage to the pelvic floor were revealed (Fig. 5).
Thirty asymptomatic parous volunteers were also studied. The average age of women enrolled in this study was 43 years, with an average of deliveries and 3.1 parturitions. Average body mass index (BMI) of volunteers was 30. Total prolapse volume in this group was 19.3 ccs, with widespread variability (2.3–260 ccs). The dynamic prolapse increment on average was 28 % (16–51 %).
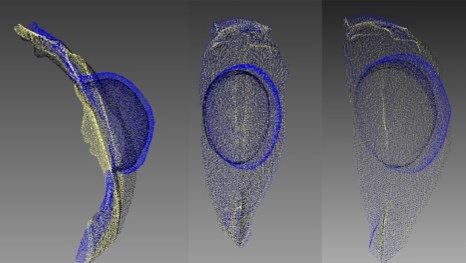
Fig. 5 Asymmetric damage to pelvic floor in a patient with pelvic organ prolapse (POP). Overlapping images at rest, during straining, and after prolapse reposition
Discussion
A new instrument for evaluating and measuring pelvic floor mobility was introduced during this study. Details are presented for creating real-time 3D models at rest and during maximal Valsalva efforts in patients with POP and in volunteers without pelvic floor disorders. In addition, an analytical method for these models was proposed.
Functional disorders of the pelvic floor represent a common health problem, especially in women. According to studies, >15 % of multiparous women have complaints of some pelvic floor disorders [6]. An association between low levels of pelvic floor knowledge and a prevalence of high pelvic floor dysfunction has been demonstrated [7]. This condition often significantly impairs quality of life and results in a variety of symptoms. Depending on the involvement of the different compartments, the spectrum of dysfunction includes urinary incontinence, prolapse, and constipation, which occur in varying combinations. Although surgical correction of a single-compartment prolapse is possible, symptomsmay recur in many patients due to recurrence in other compartments that were not simultaneously repaired.
Extensive use of synthetic materials allowed the creation of several well-standardized surgical procedures for POP correction. Nonetheless, it has become apparent that synthetic meshes may not be appropriate for all patients and can even cause severe complications when used improperly. Treating pelvic floor dysfunction has become increasingly dependent on preoperative imaging to provide an individualized, tailored approach. Dynamic imaging obtained both at rest and during straining plays an essential role in the diagnosis of pelvic floor disorders and is crucial for choosing one method of treatment over another.
The POP-Q systemwas introduced to assess the severity of POP, which is defined as the position of the pelvic floor under maximum straining. However, this method does not take into account the dynamics of the changing position of the pelvic floor mobility (rest/stress). A 2D system does not reveal true movement of the prolapsed vaginal wall and cannot identify the presence of isolated/unilateral pelvic floor defects [8]. These points play an important role in choosing the appropriate surgical technique for POP correction.
Dynamic MRI is another imaging technique that can assess pathological features of functional pelvic organs. However, this technique requires simultaneous and separate opacification of the bladder, vagina, and rectum. Furthermore, it is time consuming and invasive. MRI has gained an increasing role in assessing pelvic floor dysfunction because of its multiplanar imaging capability and the absence of ionizing radiation. Several systems of pelvic floor measurements have been proposed to improve the diagnosis of POP and to analyze the correlation between these measuring techniques and the clinical examination [9, 10]. Given that there is no standardized system for MRI measurements, studies have led to limited and noncomparable data [11]. Specialized programs have been developed to create 3D processing of the obtained MR images [12]. However, this method has some limitations:
- (a) Study duration
- (b) High cost of research
- (c) Need for long and strenuous sessions for the patient
- (d) Need for skills in 3D graphics.
A pelvic ultrasound is another diagnostic tool for evaluating POP and can be useful for detecting any structural abnormalities of pelvic floor structures, particularly levator ani muscles, and pelvic floor mobility [13, 14]. The limitation of this method is the need to reposition the prolapse, which prevents an estimate of POP severity. An ultrasound can be applied in a 3D mode; however, despite its cost effectiveness, a 3D ultrasound functional diagnostic procedure remains feasible only for well-equipped scientific research institutes and highly specialized centers.
Kruger reported a comparative study of the biometric measures of pelvic floor function obtained using 3D ultrasound and MRI in a group of young, nulliparous, asymptomatic women [15]. There was a systematic but nonsignificant difference between methods. Furthermore, both methods had substandard agreement in evaluating the area of the hiatus on Valsalva. Moreover, the main limitations of MRIand ultrasound-based 3D models remain: (a) they are based on digital computations of 2D images projecting reconstructions, and (b) they may possibly generate additional artefacts.
A cost-effective and noninvasive method was proposed during this study by creating a 3D model of the pelvic floor surface, with opportunities to measure dynamic characteristics of prolapsed tissues. Although POP stage may be similar, properties of prolapsed tissues can vary in elasticity, leading to greater enlargement of the prolapsed vaginal walls on straining. Fascial defects of the pelvic floor can lead to bladder, bowel, and uterus displacement. It remains controversial as to whether predominant deficiencies of uterine ligaments can cause severe prolapse without dislocating adjacent organs. Unawareness of the particular characteristics of supportive structures results in postoperative functional disorders, such as de novo stress incontinence, pelvic pain syndrome, dyspareunia, and constipation. Pelvic floor overcorrection by surgery is a matter requiring urgent study, as is the need for additional research evaluating normal pelvic floor mobility. The clinical sequelae of identified asymmetric pelvic floor defects also require more investigation.
Our study has both advantages and limitations. First, there was no validation process of this technique, including repeatability and reproducibility during daily activity. In addition, the possibility of suboptimal efforts of straining during Valsalva may have biased results. This was a pilot study, and the practical significance of the described method needs further investigation. The optical method employed was not designed to detect structural pathologies of the pelvic floor but to objectify pelvic floor surface movements. Thus, the described technique should be considered complementary to other diagnostic modalities, such as pelvic ultrasound or MRI.
Normal dynamic characteristics of the female pelvic floor are understudied. It is evident that the female pelvic floor is not a strictly fixed structure, as it is dynamically involved in voiding and defecation. It is an active, functional unit that has a certain degree of mobility. Data showed that the pelvic floor of asymptomatic parous women has a significant level of mobility. Determining characteristics of the pelvic floor of asymptomatic women might provide a basis for estimating their 3D models, which could be used for active surveillance. This may help predict manifestations of prolapse progression. At the same time, women with impaired elastic pelvic floor features could be identified as an at-risk group with an increased likelihood of developing pelvic floor disorders.
We demonstrated that determining pelvic floor mobility in women with POP by creating a 3D model is a valuable tool in addition to existing methods. This method is proposed for use in a tailored and personalized approach to treating pelvic floor dysfunction in clinical practice.
Conclusion
Three-dimensional scanning of the pelvic floor surface with a portable optic scanner is a valuable tool for evaluating the pelvic floor of women with pelvic disorders. Dynamic parameters of this method are useful for estimating the degree of prolapse and vaginal wall elasticity while straining. Some patients with severe prolapse showed significant enlargement in prolapse volume when engaged in physical activity, even though prolapse stage, as measured by the most prominent edge, remained the same. This information might be helpful for selecting a method of treatment by taking into consideration individual reserves of vaginal wall elasticity, thus avoiding overcorrection and functional complications. Further studies of dynamic pelvic floor features in asymptomatic women are necessary in order to implement an active surveillance program for pelvic floor disorders.
Keywords Pelvic floor mobility . Dynamic 3Dmodels . Pelvic organ prolapse
References
1. Martins JA, PatoMP, Pires EB, Jorge RM, ParenteM,Mascarenhas T (2007) Finite element studies of the deformation of the pelvic floor. Ann N Y Acad Sci 1101:316–34. doi:10.1196/annals.1389. 019
2. van Raalte H, Egorov V (2015) Characterizing female pelvic floor conditions by tactile imaging. Int Urogynecol J 26(4):607–9. doi: 10.1007/s00192-014-2549-9
3. Ren S, Xie B,Wang J, RongQ (2015) Three-dimensional modeling of the pelvic floor support systems of subjects with and without pelvic organ prolapse. Biomed Res Int 2015:845985. doi:10.1155/ 2015/845985
4. Chen L, Ashton-Miller JA, DeLancey JO (2009) A 3D finite element model of anterior vaginal wall support to evaluate mechanisms underlying cystocele formation. J Biomech 42(10):1371–7. doi:10.1016/j.jbiomech.2009.04.043
5. Ramanah R, Berger MB, Chen L, Riethmuller D, Delancey JO (2012) See it in 3D!: researchers examined structural links between the cardinal and uterosacral ligaments. Am J Obstet Gynecol 207(5):437.e1–7. doi:10.1016/j.ajog.2012.08.036
6. Olsen AL, Smith VJ, Bergstrom JO, Colling JC, Clark AL (1997) Epidemiology of surgically managed pelvic organ prolapse and urinary incontinence. Obstet Gynecol 89(4):501–6. doi:10.1016/ S0029-7844(97)00058-6
7. Berzuk K, Shay B (2015) Effect of increasing awareness of pelvic floor muscle function on pelvic floor dysfunction: a randomized controlled trial. Int Urogynecol J 26(6):837–44. doi:10.1007/ s00192-014-2599-z
8. Persu C, Chapple CR, Cauni V, Gutue S, Geavlete P (2011) Pelvic organ prolapse quantification system (POP-Q) a new era in pelvic prolapse staging. J Med Life 4(1):75–81
9. Betschart C, Chen L, Ashton-Miller JA, Delancey JO (2013) On pelvic reference lines and the MR evaluation of genital prolapse: a proposal for standardization using the pelvic inclination correction system. Int Urogynecol J 24(9):1421–8. doi:10.1007/s00192-0132100-4
10. Fauconnier A, Zareski E, Abichedid J, Bader G, Falissard B, Fritel X (2007) Dynamic magnetic resonance imaging for grading pelvic organ prolapse according to the international continence society classification: which line should be used? Neurourol Urodyn 27: 191–197
11. Lakeman MM, Zijta FM, Peringa J, Nederveen AJ, Stoker J, Roovers JP (2012) Dynamic magnetic resonance imaging to quantify pelvic organ prolapse: reliability of assessment and correlation with clinical findings and pelvic floor symptoms. Int Urogynecol J 23(11):1547–54. doi:10.1007/s00192-012-1772-5
12. Larson KA, Luo J, Guire KE, Chen L, Ashton-Miller JA, DeLancey JO (2012) 3D analysis of cystoceles using magnetic resonance imaging assessing midline, paravaginal, and apical defects. Int Urogynecol J 23(3):285–93. doi:10.1007/s00192-011-1586-x
13. Thompson JA, O’Sullivan PB, Briffa K, Neumann P, Court S (2005) Assessment of pelvic floor movement using transabdominal and transperineal ultrasound. Int Urogynecol J Pelvic Floor Dysfunct 16(4):285–92
14. Dietz HP (2011) Pelvic floor ultrasound in prolapse: what’s in it for the surgeon? Int Urogynecol J 22(10):1221–32. doi:10.1007/ s00192-011-1459-3
15. Kruger JA, Heap SW, Murphy BA, Dietz HP (2008) Pelvic floor function in nulliparous women using three-dimensional ultrasound and magnetic resonance imaging. Obstet Gynecol 111(3):631–8. doi:10.1097/AOG.0b013e3181655dc2